Hello everyone,
I hope all is well. Welcome to part 2 of this short series over Foregen's scientific work and background. This section will look at our 2018 study, which was aimed at using a decellularization process on adult human foreskin tissue, where the resulting acellular matrix could be used as a scaffold for foreskin reconstruction.
I know the last one was a bit long, but this one will be relatively short in comparison.
Foregen’s 2018 Decellularization Study
I mentioned in part 1 that many tissue decellularization methods exist [1], and which one you might use is dependent on the tissue you’re decellularizing and what your application is. The process that Dr. Bondioli and her colleagues developed and use routinely for treating burns and other types of wounds is both chemical and physical, though it is not as complicated as it seems on the surface; I’ll do my best to concisely describe the decellularization process within the context of our study, and briefly discuss the analysis methods used. This will be very surface-level, but if there is interest in a more detailed explanation, I’d be more than happy to go into more detail in the future.
Preliminary Work
It’s scarce to see living humans or donated human tissues being used in the early stages of biomedical research. For various reasons, all dealing with ethics, cost, safety, or availability, some analog or approximation is used as a stand-in; this is where in vitro, computational, and animal models usually appear.
Because this study aimed to develop a suitable tissue engineering scaffold from decellularized human foreskin tissue, the decellularization process needed to be optimized before we could even begin work with human tissue. Therefore, we did a simple preliminary study to fine-tune our method. Instead of human prepuces, we sourced bovine prepuces, which are similar enough for our purposes. The animal tissue was submitted to the decellularization process, and we then analyzed the resulting matrices to determine what the optimal protocol for human prepuce tissue would likely be.
Human Foreskin Tissue Procurement
Because our goal is the reconstruction of the human foreskin, and we seek to do that through tissue engineering principles, Foregen, therefore, occupies a peculiar position. A relatively common practice in regenerative medicine and bioengineering is the utilization of infant foreskin tissue, which is obtained from routine circumcision discards. In addition to being one of the few sources of readily available and inexpensive human tissue, this young tissue has particular properties that give it an astoundingly high level of utility. For instance, one of the most prominent examples is artificial skin replacements, which are produced using fibroblast cells isolated from the foreskin tissue [2].
Now, if you're reading this, I imagine you already know where this is going. Late last year, I posted my lengthy ethics preprint, where I provided a meticulous analysis of the morality of this practice; I argue that non-therapeutic circumcision of children is partial murder and theft of their bodies, and the discarded tissue's use in biotechnology constitutes wrongful use, which morally taints any of the subsequent work. I conclude that, as a whole, the practice is immensely unethical, as it relies on the violent exploitation of children, and biotechnology absolutely must abandon the practice [3].
Admittedly, the road towards Foregen's goal would be quicker, easier, and less costly if we opted to use neonatal foreskin tissue in our work. However, we all have made an ironclad commitment to never engage in or support this ignominious practice. It is the most significant and pernicious of errors to assert that this world has only a physical and not a moral significance [4]. Therefore, we must be mindful of the moral qualities of our actions, as ethics demands that we make our actions right, which is to say that they do not infringe against justice [5]. Adults that are sound-of-mind may consent to the donation of parts of their body for whatever reason, and no wrong is committed. This is due to the self-evident principle "No injury is done to someone who wills it [volenti non fit injuria]: for since what I do is at all times what I will, what happens to me from myself too is always only what I will, and consequently never a wrong." Moreover, adults can arrange to donate their remains after death. However, children differ from adults because they are not mature in their judgment. Even if a child consented to donate their foreskin specifically for our research, we would find that action infringes against justice and what is right, as the principle "no injury is done to him who wills it" cannot be invoked [6]. Although an argument can be made that it's permissible to use neonatal tissue when medical circumcision is warranted, infant foreskin tissue obtained through non-therapeutic circumcisions will always be morally wrong and will taint the work that uses it.
Being that I am both a moral philosopher as well as an engineer, I can categorically say that science and ethics are, in fact, two sides of the same coin and cannot be separated. Unfortunately, in much scientific research, ethics requirements are often treated as merely a formality, as many, even well-meaning researchers, don't understand the relationship between the two disciplines. The routine, non-therapeutic circumcision of children is a phenomenon, though convoluted in its origins and why it manifests. It is primarily a disregard of ethical principles on multiple fronts. Everyone involved with Foregen understands the connection between science and ethics and, as such, goes to more extraordinary lengths than what is typical to ensure that every aspect of our work is as moral as humanly possible. All of that said, we only will source tissue from cadaveric sources or from informed adults undergoing either medical or elective circumcision, which is how we procured the adult donor foreskin tissue for this study.
Decellularization
The decellularization method is relatively straightforward and uses trypsin, an enzyme that the pancreas secretes that has its native function in protein digestion [7]. Once our lab procured the donor tissue, the epidermal and dermal layers were separated using trypsin. They were then sectioned into two pieces, which served as experimental and control samples. The experimental samples were then decellularized using a diluted trypsin solution while on an agitation device. After 24 hours, the decellularization process was considered complete, and the resulting matrices were rinsed of the trypsin and then analyzed.
Analysis
I will do my best to keep this section brief, though substantive still, because I could provide a large amount of information on how they work for almost each of the assays that were used. Like I’ve said before, if there is interest in that, I’d be more than happy to do that, though.
The purpose of these assays was to ensure that the decellularization method is adequate for developing a scaffold for tissue engineering purposes. If we remember what I said before, the requirements for this are that the donor cells are removed, and the mechanical, structural, and chemical characteristics are preserved, which is what each of the assays served to do.
Microbiological Analysis
This assay was to ensure the sterility of the samples. The sterility ensures that the decellularization process removes any potential microbial contaminants that could compromise the quality of the matrices, which would introduce unnecessary error into the results. Sections of the samples were placed on growth media plates and incubated to check for any bacterial or fungi growth.
Histological Processing & Analysis
Samples from both experimental and controls were sectioned, and the histology was studied. We've discussed what histology is before, which is the study of tissues and their cellular organizations. This is done through staining tissues and exploring through a microscope. For our purposes, it allowed us to examine the structural characteristics of the foreskin tissue before and after decellularization.
Image Analysis
Though I was not able to assist in any of the wet bench work, due to being on a different continent, in addition to writing the text and publishing the article itself, I was able to assist with other skills of mine. Using computer imaging software, I used a method called color deconvolution, which takes advantage of the different colors of the stained tissue in histology images. The way it works can become very convoluted. Still, it allowed us to verify that specific ECM biomolecules were preserved and not washed out during decellularization.
Cell Viability Assay
This one is pretty self-explanatory, though, much like the last subsection, the way it works can be a bit complicated. It works using spectrophotometry, a prevalent technique involving shining a bright light through an experimental sample. A detector on the other side of the sample measures the amount of light that passes through. The amount of light absorbed by the sample is proportional to the concentration of the material of interest (Figure 1).

This is formally described by the Beer-Lambert Law, which says that absorbance (A) is proportional to molar absorptivity (ɑ), length of light path (L), and a solution’s concentration (C):

This simple relationship means that it is therefore very easy to solve for concentration, hence why this principle is used widely in biomedical instrumentation [8].
This principle can be used to measure the concentration of cells, or, for decellularized tissues, the lack thereof, which is how we verified the decellularization process removed the donor cells from the matrix.
Measurement of Basic Fibroblast Growth Factor
Having a protein matrix is not necessarily enough to engineer a tissue, as there need to be regulatory cues present to instruct the new cells on what to do, such as migrate, proliferate, and differentiate. One way these cues occur is as chemical signals, which are embedded in the matrix. These characteristics are grouped into the concept of bioactivity, which can be thought of as the magnitude of interaction that cells will have with a given biomaterial (we will discuss this in more detail in part 3). Generally speaking, it's desirable for the scaffold to have a high degree of bioactivity, as it is necessary for proper integration. A peculiar feature of this decellularization process is that it generates a large amount of Basic Fibroblast Growth Factor (FGFb), which was measured using the same spectrophotometry method used in the previous assay.
Mechanical Properties Analysis
Lastly, we analyzed the mechanical properties of the decellularized matrices. There exist many different tests that measure a variety of different mechanical properties. We used one of the more common tests, which was tensile testing. This involves pulling a sample in two directions until it eventually fractures (breaks). We measured four specific material properties of the foreskin samples: maximum load, tensile strength, Young’s modulus of elasticity, and stiffness. To understand what these mean, we need to dip back into materials science for a bit and get acquainted with the two parameters: engineering stress (σ) and engineering strain (ε).
Formally, engineering stress is defined as
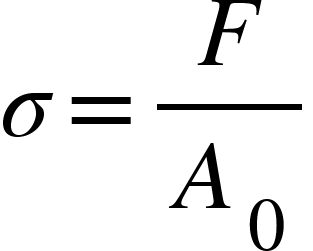
where F is the force applied perpendicular to the cross-section of a sample at any point during tensile testing, and A₀ is the original cross-sectional area of the sample. Engineering strain is defined as

where l₀ is the length of a sample before loading (before strain is applied), and lᵢ is the sample length at any point during the tensile testing procedure. When materials are strained, they can experience two types of deformation: elastic and plastic. Elastic deformation is non-permanent deformation, which is to say that when stress is removed from a material, it will return to its original shape. Plastic deformation, on the other hand, is permanent, and if strain increases, the material will eventually fracture [9]. Everyone has likely experienced this behavior in plastics or in things like rubber bands: a small amount of stretching and the band will return to its original shape, but if it's stretched too much, it will not return to its original form.
When a material is undergoing elastic deformation, stress and strain are proportional and follow a linear relationship, which is described by Hooke's law
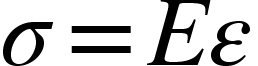
where E is Young's modulus of elasticity. On the molecular level, elastic deformation results from small changes in atomic spacing and the stretching of chemical bonds. The resistance to deformation results from these interatomic bonding forces that "pull" against the force being imparted on them, much like a spring behaves:

From this, we can say that those materials with stronger bonds will be less easily deformed. Materials with a high E are very stiff. The mechanical properties of polymers, in particular, are highly direction-dependent, as the orientation of their chains will dictate how easy they are to deform [9]. Furthermore, if we think back to part 1 of this series, I mentioned that a polymer's properties could change significantly based upon crosslink-density and molecular weight. I also said having used (polyethylene) glycol (PEG) quite a bit. The lab I worked in typically used it as a hydrogel. Based upon the crosslink-density and the molecular weight PEG we used, you could see very different types of gels after polymerization. Often, they would be soft and squishy, much like a gelatin dessert. But we could also produce gels so stiff that they were brittle like ceramics. The different types of polymers' behavior in response to tensile deformation can be visualized in the stress-strain plot in Figure 2.

We subjected donor tissue and the decellularized matrices to tensile testing using this materials science theory and obtained several critical mechanical properties. From Figure 2, we can also get a rough sense of what these other measurements mean. Stiffness is merely the slope of the curve in the elastic range. And the ultimate tensile strength of the material is simply the maximum amount of stress that can be imparted upon the material.
There's much more about the mechanical properties of materials that I did not cover. Still, those interested in learning more should check out the site Materials Science & Engineering Student because their section on mechanical properties is excellent and easy to follow.
Conclusion
What was found after each of these tests was that the decellularization process was adequate for our purposes: the process removed the previous donor cells while at the same time preserving the structural, chemical, and mechanical properties necessary for its use in tissue engineering applications. In addition to meeting these requirements, the decellularized scaffolds carry a much higher level of bioactivity, which indicates a high potential for regenerative capabilities.
We will leave things here for today, as we are now ready to consider biocompatibility and Foregen’s rat model.
References
[1] K. Bush, A.A. Gertzman, Process Development and Manufacturing of Human and Animal Acellular Dermal Matrices, in: J.H. Holmes, M. Albanna (Eds.), Ski. Tissue Eng. Regen. Med., Academic Press, London, UK, 2016: pp. 83–108. https://doi.org/10.1016/B978-0-12-801654-1.00005-X.
[2] M.L. Shuler, F. Kargi, M. DeLisa, Medical Applications of Bioprocess Engineering, in: Bioprocess Eng. Basic Concepts, 3rd ed., Prentice Hall, 2017: pp. 519–533.
[3] E.J. Cunningham, Ought Implies Can: A Kantian Analysis of the Morality of the Use of Neonatal Tissue in Biotechnology (Version 4), Zenodo. (2021). https://doi.org/10.5281/zenodo.6394406.
[4] A. Schopenhauer, On ethics, in: A. del Caro, C. Janaway (Eds.), Parerga Und Paralipomena [Parerga Paralipomena], Cambridge University Press, Cambridge, UK, 2015: pp. 183–216.
[5] I. Kant, Die Metaphysik der Sitten [The Metaphysics of Morals], Revised ed, Cambridge University Press, Cambridge, UK, 2020.
[6] A. Schopenhauer, Über die Grundlage der Moral [On the Basis of Morality], in: C. Janaway (Ed.), Die Beiden Grundprobleme Der Ethik [The Two Fundam. Probl. Ethics], Cambridge University Press, Cambridge, UK, 2009: pp. 113–258.
[7] J. Feher, Digestion and Absorption of the Macronutrients, in: Quant. Hum. Physiol. An Introd., 2nd ed., Elsevier, 2017: pp. 821–833. https://doi.org/10.1016/B978-0-12-800883-6.00081-1.
[8] L.A. Wheeler, Clinical Laboratory Instrumentation, in: J.G. Webster (Ed.), Med. Instrum. Appl. Des., 4th ed., John Wiley & Sons, Inc., Hoboken, NJ, 2010: pp. 498–527.
[9] J.S. Temenoff, A.G. Mikos, Mechanical Properties of Biomaterials, in: Biomater. Intersect. Biol. Mater. Sci., Pearson Education, Inc., Upper Saddle River, NJ, 2008: pp. 131–176.